Research Highlight #6
Origins of Hot Jupiters from the Stellar Obliquity Distribution
(Rice, Wang, & Laughlin 2022 ApJL 926, L17)AAS Journal Author Series video
Yale Scientific Magazine article
In the 90s, the discovery of the first exoplanets came as a surprise: the first confirmed exoplanet detections were massive planets orbiting shockingly close to their host stars, known as "hot Jupiters". But how do these bizarre planets — unlike anything observed in the solar system — form? This mystery has remained at the core of exoplanet science over the past three decades.
Observations of hot Jupiters' spin-orbit angles, or their "stellar obliquities" (the angle between the stellar spin axis and the hot Jupiter's orbit normal) provide a clue to their key formation pathway. Many hot Jupiters orbit their host star sideways (on polar orbits), or possibly even backwards. Some mechanisms that can produce these large hot Jupiter spin-orbit misalignments are shown below.
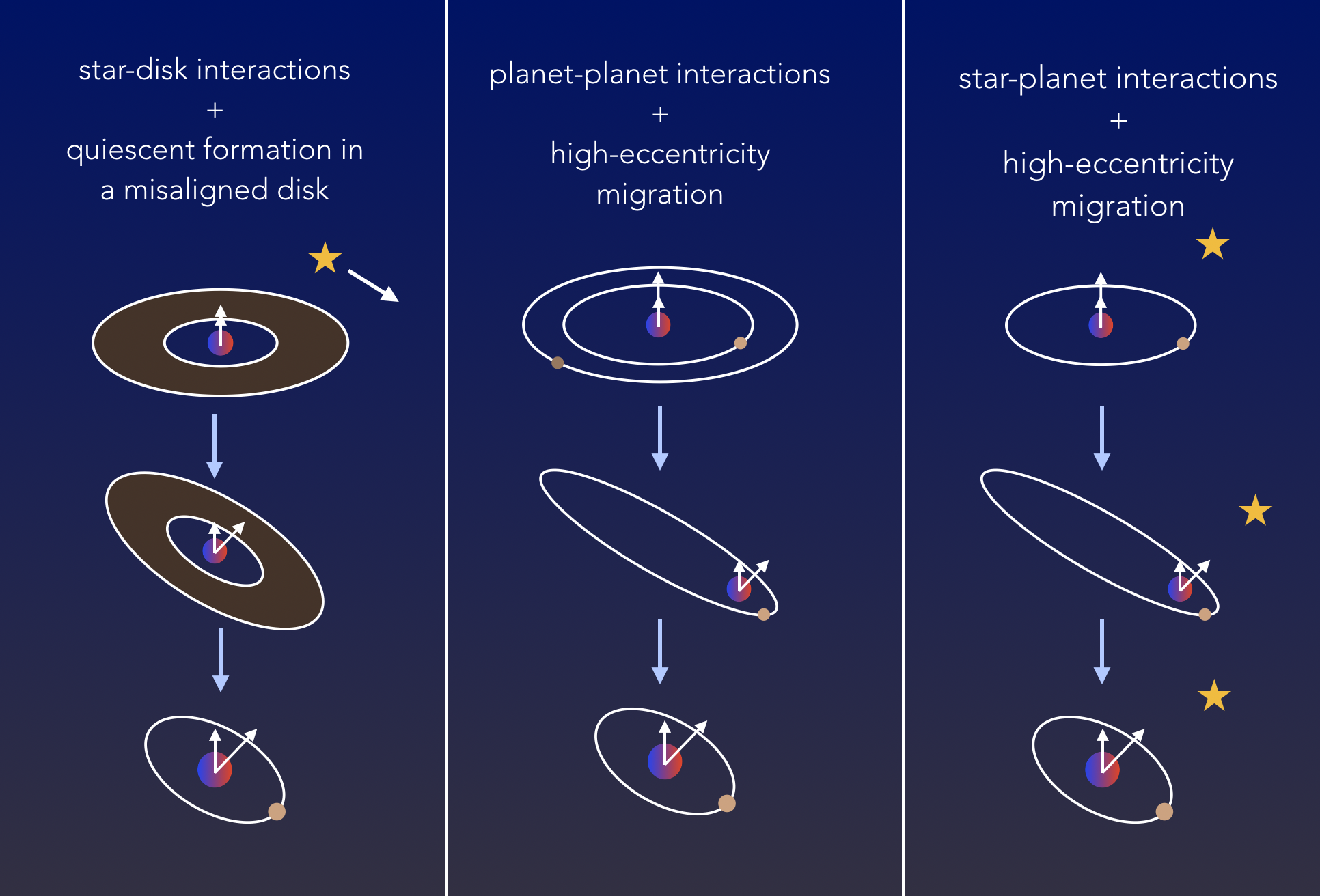
Figure 1: A few potential hot Jupiter origin channels that can produce spin-orbit misalignments.
Not all hot Jupiters show these spin-orbit misalignments; in addition to the large misaligned population, many hot Jupiters have also been found at or near alignment. Previous work (Winn et al. 2010, Schlaufman et al. 2010) demonstrated that hot Jupiters orbiting hot stars span a wide range of misalignments, while those orbiting cool stars are typically aligned. This is likely due to differences in the properties of systems with host stars above and below the "Kraft break", which is located around Teff~6100 K. Stars above the Kraft break have radiative exteriors and relatively weak tidal and magnetic interactions with their companions, while stars below the Kraft break have convective exteriors and are more capable of tidally realigning planets that had been initially misaligned.
In this paper, we add another layer to this story: that is, we show that the established discontinuity of stellar obliquities at the Kraft break is present only for hot Jupiters on circular orbits. By contrast, hot Jupiters on eccentric orbits show no significant population-wide change in stellar obliquities at the Kraft break, as demonstrated in the figure below.
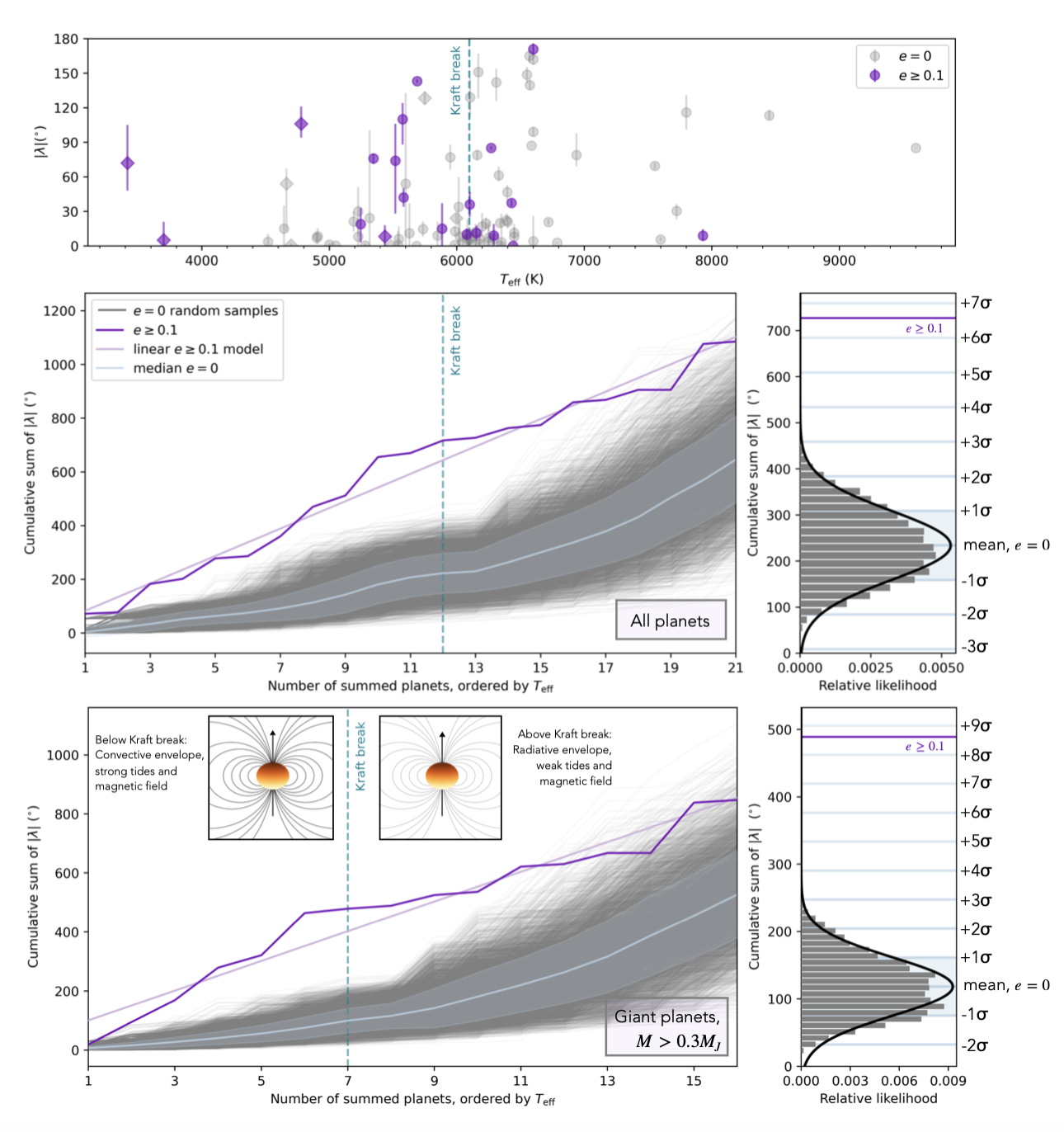
Figure 2: Comparative cumulative sums demonstrating that the difference in stellar obliquities below and above the Kraft break is not present in the population of eccentric planets.
What does this mean? Well, it may be that the planets that reached the highest eccentricities have already tidally circularized and tidally realigned, while those that are currently observed with eccentric orbits have not had time to complete these joint processes. In this case, a large fraction of hot Jupiters may have begun with large misalignments — as would be expected in the high-eccentricity migration scenario — and many such systems were subsequently realigned. We demonstrate below that the hot Jupiters with the longest tidal realignment timescales have the longest eccentricity damping timescales, as well as the largest range of measured stellar obliquities.

Figure 3: Comparison of tidal realignment timescales, eccentricity damping timescales, and sky-projected stellar obliquities.
Based on these findings, we show that the stellar obliquity distribution of hot Jupiters is consistent with being crafted by a combination of high-eccentricity migration and tidal dissipation. In this framework, most or all hot Jupiters originated further out in their host protoplanetary disks. Dynamical interactions launched these cold Jupiters to extremely high eccentricities and a wide range of misalignments, and the orbits were subsequently circularized and realigned by tidal interactions between the planet and its host star. The current stellar obliquity distribution reflects the natural tidal realignment process that acts most efficiently for planets orbiting cool stars.

Figure 4: Observed stellar obliquity distribution, divided into four subsets, overlaid with theoretical distributions.
The difference at the Kraft break is also visualized above. Only the hot Jupiter population with circular orbits and cool host stars shows a significant excess of aligned planets, while the two eccentric populations overlap. In gray, we overplot fiducial stellar obliquity distributions that result from high-eccentricity migration pathways. These overlap well with all populations other than the e=0 hot Jupiters orbiting cool stars, suggesting that these planets may have been realigned, while the other three populations have not been as strongly affected by tidal dissipation.
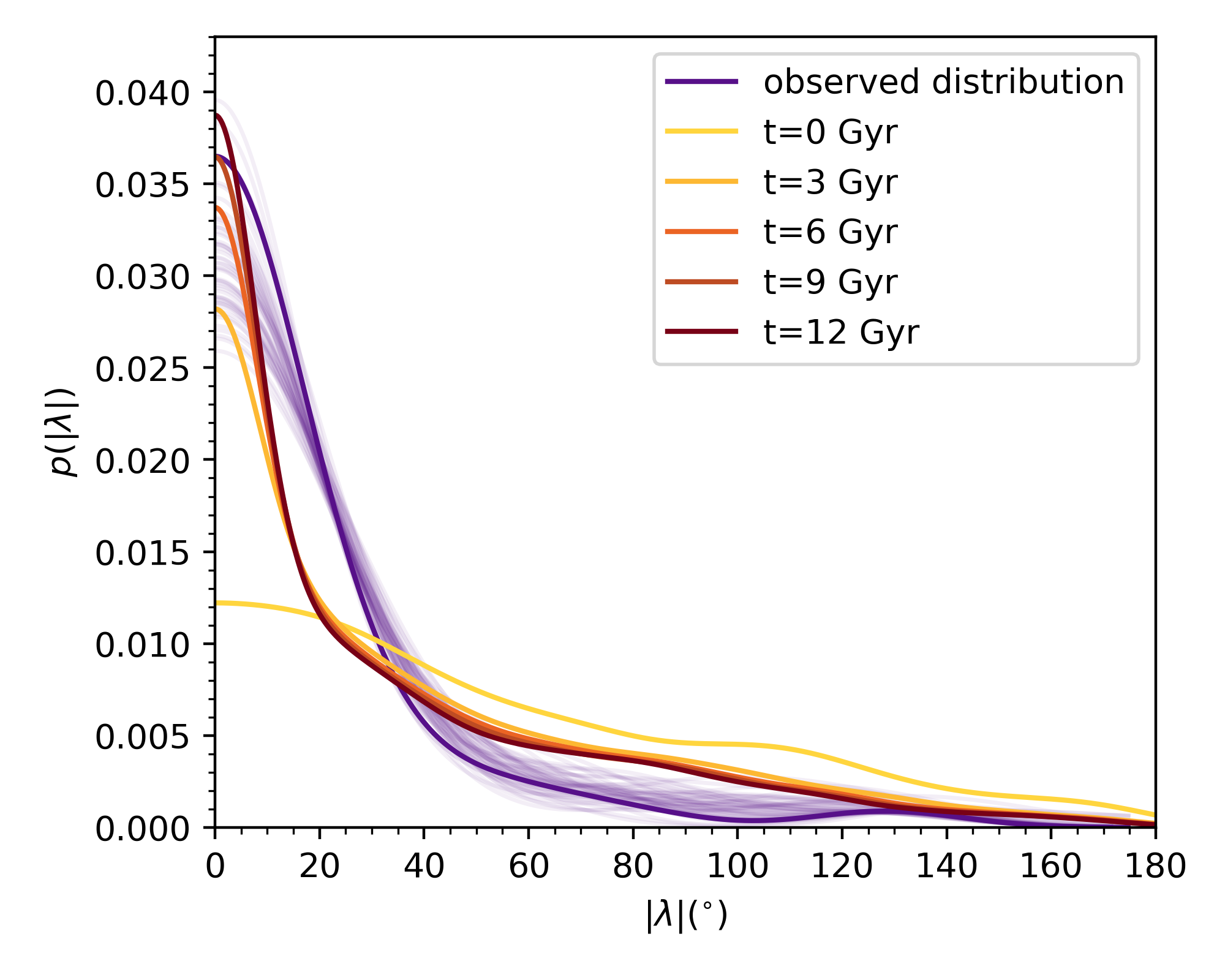
Figure 5: Evolution of hot Jupiter stellar obliquities in a high-eccentricity migration mixture model.
Finally, we evolve a mixture model of the high-eccentricity migration pathways shown above to demonstrate that high-eccentricity migration, combined with tidal dissipation, can reproduce the global properties of the stellar obliquity distribution. Our analysis provides a tantalizing hint that high-eccentricity migration may be a (the?) dominant hot Jupiter formation mechanism, pushing us one step closer to answering the long-standing question of how hot Jupiters are created.